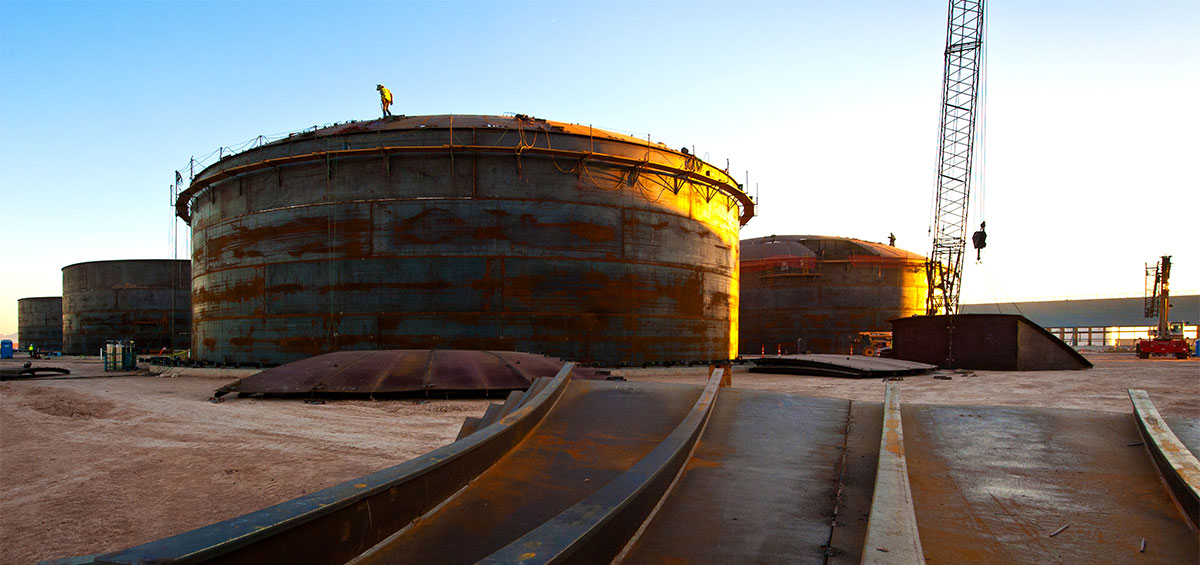
Unlock the power of sustainable energy management with thermal energy storage (TES), revolutionizing how we store, manage, and utilize heat energy for a brighter, more efficient future.
What is thermal energy storage?
Thermal energy storage (TES) is a technology that allows for the storage of thermal energy in various forms for later use. It enables the shifting of energy demand from peak to off-peak hours and helps to better match energy supply with demand.
The advantages of thermal energy storage
Thermal energy storage (TES) offers several advantages across various sectors and applications:
- Energy Flexibility: TES systems allow for the storage of excess energy generated during off-peak hours for later use during peak demand periods. This flexibility helps to better match energy supply with demand, improving grid stability and reducing the need for additional energy generation capacity.
- Energy Efficiency: By storing thermal energy for later use, TES systems can improve overall energy efficiency in various applications. For example, in HVAC systems, storing excess thermal energy during off-peak hours allows buildings to reduce their reliance on energy-intensive cooling or heating systems during peak demand periods.
- Cost Savings: TES systems can help reduce energy costs by allowing users to take advantage of off-peak electricity rates for charging the storage systems. By storing energy when electricity prices are low and using it during peak demand periods when prices are high, users can save on their energy bills.
- Integration of Renewable Energy: TES systems play a crucial role in the integration of renewable energy sources, such as solar and wind power, into the grid. These sources often generate energy intermittently, but TES allows for the storage of excess energy generated during sunny or windy periods for later use when energy production is lower.
- Grid Stability: TES systems can contribute to grid stability by providing a means to store excess energy and balance fluctuations in supply and demand. This helps to reduce the need for backup power plants and grid infrastructure upgrades to meet peak demand periods.
- Reduced Environmental Impact: By enabling the integration of renewable energy sources and improving energy efficiency, TES systems can help reduce greenhouse gas emissions and mitigate environmental impact associated with traditional energy generation methods.
- Load Shifting: TES systems enable load shifting, which involves moving energy consumption from peak to off-peak hours. This helps to alleviate strain on the grid during peak demand periods and reduces the risk of blackouts or brownouts.
- Enhanced Resilience: TES systems can improve the resilience of energy systems by providing backup energy storage during power outages or emergencies. In applications such as microgrids or off-grid systems, TES can ensure a reliable energy supply even in challenging conditions.
What are the ways to store thermal energy?
There are several methods to store thermal energy, each with its own advantages and applications:
- Sensible Heat Storage: This method involves storing thermal energy by changing the temperature of a material. Common materials used for sensible heat storage include water, rocks, and bricks. Thermal energy is added to the material to raise its temperature or removed to lower its temperature. Sensible heat storage systems are relatively simple and cost-effective. They are commonly used in solar water heaters, district heating systems, and some HVAC applications.
- Latent Heat Storage: Latent heat storage involves storing thermal energy by changing the phase of a material, typically from solid to liquid or vice versa. During the phase change, the material absorbs or releases a large amount of energy without a significant change in temperature. Phase change materials (PCMs) are commonly used for latent heat storage. PCMs include substances like paraffin wax, salt hydrates, and certain organic compounds. Latent heat storage systems are used in applications where maintaining a constant temperature is important, such as in building insulation, refrigeration, and thermal energy storage for solar power plants.
- Thermochemical Storage: Thermochemical storage involves storing thermal energy by triggering a reversible chemical reaction that absorbs or releases heat. During charging, the reactants absorb heat and undergo a chemical transformation, storing the energy. During discharging, the reverse reaction occurs, releasing the stored heat. Thermochemical storage systems typically use a pair of chemical compounds that can undergo reversible reactions. Thermochemical storage is still in the research and development stage but shows promise for high-density and long-duration energy storage applications.
- Molten Salt Storage: Molten salt storage involves heating a mixture of molten salts to a high temperature to store thermal energy. Molten salt storage systems are commonly used in concentrating solar power (CSP) plants to store excess heat generated by solar thermal collectors during the day for electricity generation at night or during cloudy periods. Molten salt storage systems can provide high-temperature thermal energy storage with relatively high energy density and efficiency.
- Aquifer Thermal Energy Storage (ATES): ATES involves storing thermal energy in underground aquifers by injecting or extracting water from wells. During the heating season, hot water is injected into the aquifer, where it heats up the surrounding rock and water. During the cooling season, cold water is injected into the aquifer to extract heat from buildings or industrial processes. ATES systems are used for seasonal thermal energy storage in buildings, district heating and cooling systems, and greenhouse operations.
What is pit thermal energy storage?
Pit thermal energy storage (PTES) is a form of seasonal thermal energy storage (STES) that involves storing excess thermal energy in the ground during warmer months and extracting it for use during colder months. It is a sustainable and cost-effective method of storing thermal energy for heating and cooling purposes in buildings, district heating systems, and industrial processes.
In a pit thermal energy storage system, a large pit or underground chamber is excavated and lined with a suitable impermeable material to prevent leakage. The pit is then filled with a storage medium, such as water or a mixture of water and antifreeze, which acts as the heat transfer fluid. Alternatively, pipes or tubes containing the heat transfer fluid may be buried in the pit.
During the summer or warmer months, excess thermal energy from sources such as solar collectors, waste heat from industrial processes, or heat pumps is used to heat the storage medium in the pit. The heat is transferred to the ground, raising the temperature of the soil or rock surrounding the pit.
In the winter or colder months, the stored thermal energy is extracted from the ground by circulating the heat transfer fluid through the pit or buried pipes. The heat is then transferred to buildings, district heating networks, or other heat consumers for space heating or hot water production.
Pit thermal energy storage offers several advantages, including:
- Renewable Energy Integration: PTES systems can store excess thermal energy from renewable sources such as solar thermal collectors or heat pumps, allowing for better integration of renewable energy into heating and cooling systems.
- Cost-Effectiveness: PTES systems can reduce energy costs by allowing buildings and facilities to rely on stored thermal energy during peak demand periods or when energy prices are high.
- Space Savings: Compared to above-ground thermal energy storage systems, PTES systems require less surface area, making them suitable for urban areas or locations where space is limited.
- Low Environmental Impact: PTES systems have minimal environmental impact and can contribute to reducing greenhouse gas emissions by displacing fossil fuel-based heating and cooling systems.
- Long-Term Storage: PTES systems can provide seasonal thermal energy storage, allowing for the storage of thermal energy for several months or even years.
How pit thermal energy storages are constructed
Constructing a pit thermal energy storage (PTES) system involves several steps to excavate, prepare, and equip the pit for storing and extracting thermal energy. Here is a general outline of the construction process:
- Site Selection and Preparation:
- Choose a suitable location for the PTES system, considering factors such as soil type, groundwater level, and proximity to heat sources and consumers.
- Obtain necessary permits and approvals from local authorities and regulatory agencies.
- Clear the site of any vegetation, debris, or obstacles that may interfere with excavation and construction activities.
- Excavation:
- Excavate a pit or underground chamber large enough to accommodate the desired storage capacity and depth. The size and shape of the pit may vary depending on site-specific conditions and project requirements.
- Ensure proper slope and stability of the excavation walls to prevent collapse or soil erosion.
- Lining and Insulation:
- Line the walls and floor of the pit with a suitable impermeable material, such as geomembrane or concrete, to prevent leakage of the storage medium.
- Install insulation material around the perimeter of the pit to minimize heat losses to the surrounding soil and improve the overall efficiency of the PTES system.
- Storage Medium Installation:
- Fill the pit with a storage medium, such as water or a mixture of water and antifreeze, which will act as the heat transfer fluid.
- Alternatively, install pipes or tubes containing the heat transfer fluid in the pit or buried beneath the ground surface. These pipes will circulate the fluid to absorb or release thermal energy as needed.
- Pump and Control System Installation:
- Install pumps, valves, and control systems to circulate the heat transfer fluid between the pit and heat sources/consumers.
- Connect the PTES system to heat sources, such as solar thermal collectors, waste heat recovery systems, or heat pumps, as well as heat consumers, such as buildings or district heating networks.
- Testing and Commissioning:
- Conduct tests to ensure the integrity and functionality of the PTES system, including pressure tests, leak detection, and performance evaluations.
- Fine-tune control parameters and operational settings to optimize the performance and efficiency of the system.
- Operation and Maintenance:
- Regularly monitor and maintain the PTES system to ensure proper operation and performance.
- Inspect the pit, storage medium, and associated components for signs of wear, corrosion, or damage.
- Perform routine maintenance tasks, such as cleaning filters, lubricating moving parts, and checking fluid levels and pressures.
Using geosynthetics in pit heat energy storage
Geosynthetic products can be utilized in pit thermal energy storage (PTES) systems to enhance the performance, durability, and efficiency of the storage facility. Here are several ways geosynthetics can be integrated into PTES construction:
- Geomembrane Liners: Geomembranes are impermeable synthetic liners commonly used to line the walls and floor of PTES pits to prevent leakage of the storage medium (e.g., water or antifreeze solution). Geomembranes provide a reliable barrier against fluid migration into or out of the storage pit, ensuring the integrity of the system and preventing contamination of surrounding soil and groundwater.
- Geotextile Protection: Geotextiles can be used to protect geomembranes from damage during installation and operation. Placing a geotextile layer between the geomembrane liner and the backfill material helps to cushion the liner against punctures, abrasion, and mechanical stresses caused by rocks, roots, or other sharp objects. Geotextiles also promote drainage and filtration to prevent the buildup of hydrostatic pressure behind the liner.
- Geocomposite Drainage Layers: Geocomposite drainage layers consisting of a combination of geotextiles and drainage cores can be installed above or below the geomembrane liner to facilitate the removal of excess water or condensation from the storage pit. By providing efficient drainage and moisture management, geocomposite layers help to maintain stable soil conditions, minimize the risk of liner damage, and enhance the long-term performance of the PTES system.
- Geogrid Reinforcement: In cases where the soil surrounding the PTES pit exhibits poor stability or bearing capacity, geogrids can be used to reinforce the backfill material and improve overall structural integrity. Geogrids are high-strength synthetic grids that are placed within the soil mass to distribute loads, reduce settlement, and enhance load-bearing capacity. By stabilizing the surrounding soil, geogrid reinforcement ensures the stability and longevity of the PTES pit.
- Geocomposite Gas Venting Systems: Geocomposite gas venting systems consisting of geotextile filters and drainage cores can be installed within the backfill material to facilitate the venting and extraction of gases generated within the storage pit, such as methane or carbon dioxide. By promoting gas diffusion and venting, geocomposite gas venting systems help to mitigate the risk of gas buildup, prevent pressure buildup, and maintain a safe and stable environment within the PTES pit.
Conclusion
In conclusion, thermal energy storage (TES) offers a versatile solution for managing energy demand, integrating renewable sources, and enhancing energy efficiency. By storing excess thermal energy for later use, TES systems contribute to grid stability, cost savings, and environmental sustainability across various sectors.